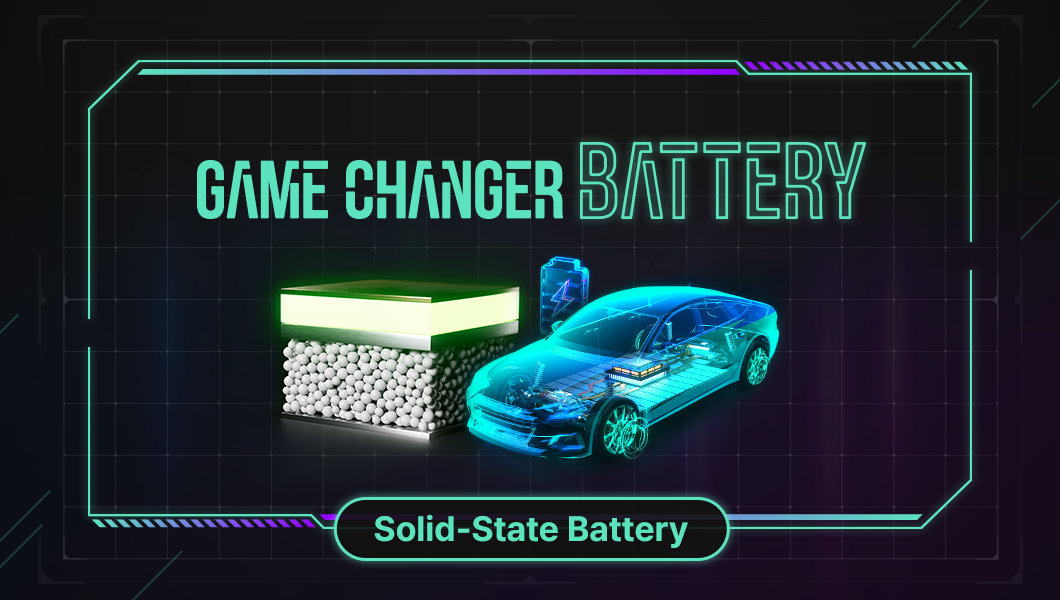
We are living in the age of batteries. The secondary battery market is expanding rapidly, and public interest in the next level of secondary batteries is growing.
The battery industry is especially focusing on all-solid-state batteries, which are known for their high safety and outstanding energy density. Experts expect that all-solid-state batteries will overcome the theoretical limitations of lithium-ion batteries, elevating battery technology to a higher level.
Today, let’s dive into all-solid-state batteries, which are moving forward to overcome the limitations of lithium-ion batteries.
What is an All-Solid-State Battery?

A lithium-ion battery consists of a cathode, an anode, an electrolyte, and a separator. Among them, the electrolyte, which serves as the pathway for lithium ions, is typically made of a liquid with high ionic conductivity. An all-solid-state battery is a secondary battery that replaces this liquid electrolyte with a solid one. Accordingly, all components in an all-solid-state battery are solid. Notably, the solid electrolyte itself can act as a separator, as it is solid.
All-Solid-State Batteries, Boasting Excellent Safety and High Energy Density
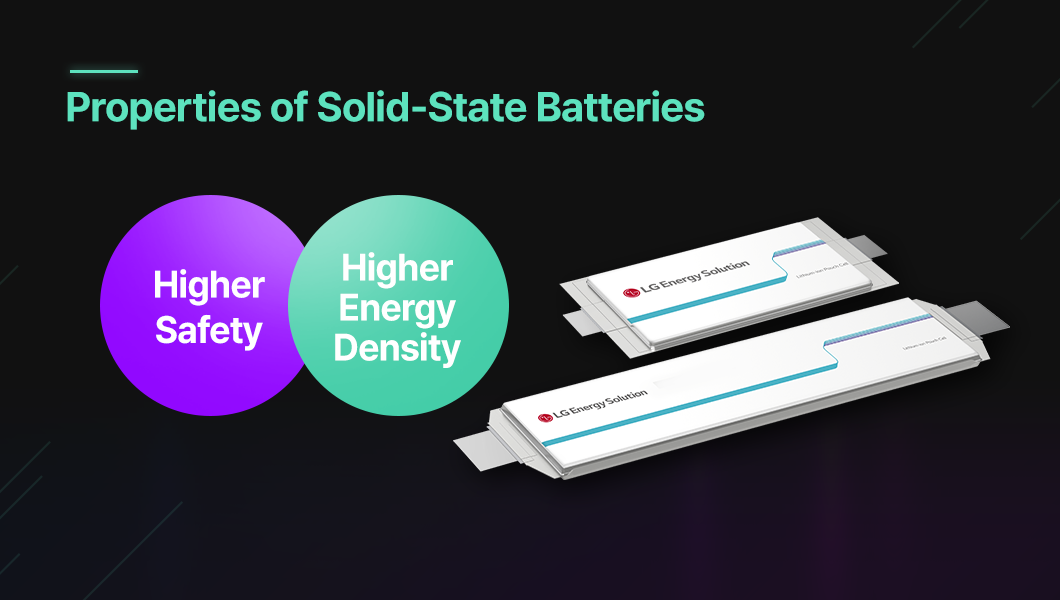
The key feature of all-solid-state batteries is their “excellent safety.” Since the electrolyte is solid, there is no risk of leakage due to external shocks, and its high thermal stability helps eliminate the risk of fire.
All-solid-state batteries can also offer “high energy density*.” Solids basically have higher density than liquids of the same volume. In addition to that, by replacing the liquid electrolyte with a solid one, the battery can eliminate the separator and instead add more anode and cathode active materials, thereby increasing energy density. Moreover, the enhanced safety of these batteries means that heat management systems, which normally take up much space, can be reduced, allowing more cells to be put into the battery pack. This directly translates to an increased driving range for electric vehicles.
*Energy Density: The amount of energy per unit weight or volume.
Additionally, the solid electrolyte is less affected by external temperature compared to liquid electrolytes, which helps alleviate the performance degradation that often occurs in batteries during winter.
Types of Solid Electrolytes
The main solid electrolytes currently under research for all-solid-state batteries are “Sulfide,” “Oxide,” and “Polymer” types.
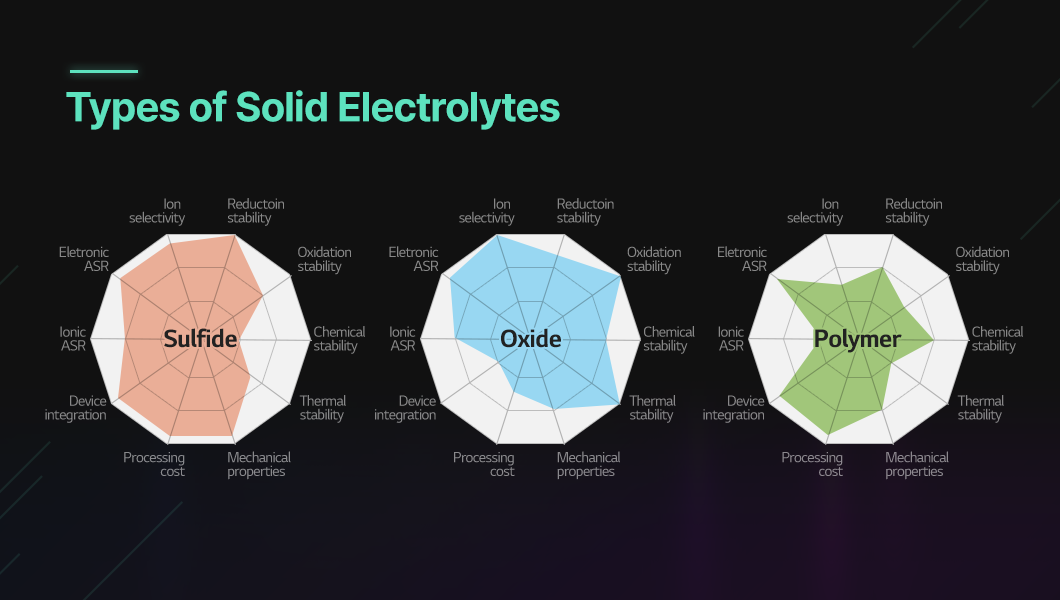
■ Sulfide: This material forms a broad interface between the electrode and the electrolyte, resulting in the highest conductivity among the three and uniform interfacial resistance, making it the most promising solid electrolyte. However, it produces hydrogen sulfide (H2S) during the manufacturing process and is sensitive to moisture, requiring strict moisture control throughout the process.
*Interface: The boundary formed between any two phases (gas, liquid, solid) when they come into contact.
■ Oxide: Although it has lower ionic conductivity than sulfides, it demonstrates excellent electrochemical stability in air. However, due to difficulties in forming a contact interface between the electrolyte and the electrode, it should be treated at very high temperatures for synthesis.
■ Polymer: This hybrid form is compatible with various types of electrolytes, such as liquid or solid, and can leverage the currently used liquid electrolyte production processes, reducing processing costs. However, compared to sulfide/oxide-based materials, its lower ionic conductivity can lead to reduced performance and output at low temperatures, necessitating continued research.
Anode Materials Used in All-Solid-State Batteries
Research is underway on using “Lithium Metal Anodes” and “Silicon Anodes” to enhance the energy density and commercialization of all-solid-state batteries.
■ Lithium Metal Anodes: Utilizing lithium metal as an anode material can result in the formation of dendrites* on the anode surface, which can reduce battery capacity and charging efficiency. However, the solid electrolyte in all-solid-state batteries plays a robust role as a separator, helping to suppress cell damage caused by dendrites. By applying lithium metal anodes, the capacity can be increased by about 10 times compared to using graphite anodes, as the lithium metal anode can store more lithium ions coming from the cathode. Consequently, the energy density can be significantly improved. Also, the lithium ions moving to the anode are reduced to lithium metal immediately, which accelerates battery charging speed.
*Dendrite: Lithium crystals formed on the anode surface during battery charging/discharging.
■ Silicon Anodes: Silicon anodes, which have much higher energy density compared to the commonly used graphite anodes in lithium-ion batteries, can be applied to all-solid-state batteries. Due to their higher theoretical capacity*, silicon anodes are considered essential for improving battery energy density. However, their volume can change significantly during charging and discharging, which is why research continues to find a way for increasing the silicon content in anodes.
*Theoretical Capacity: The maximum lithium-ion content that can be stored within an electrode material (referenced from the Korean Vacuum Society).
Challenges of All-Solid-State Batteries
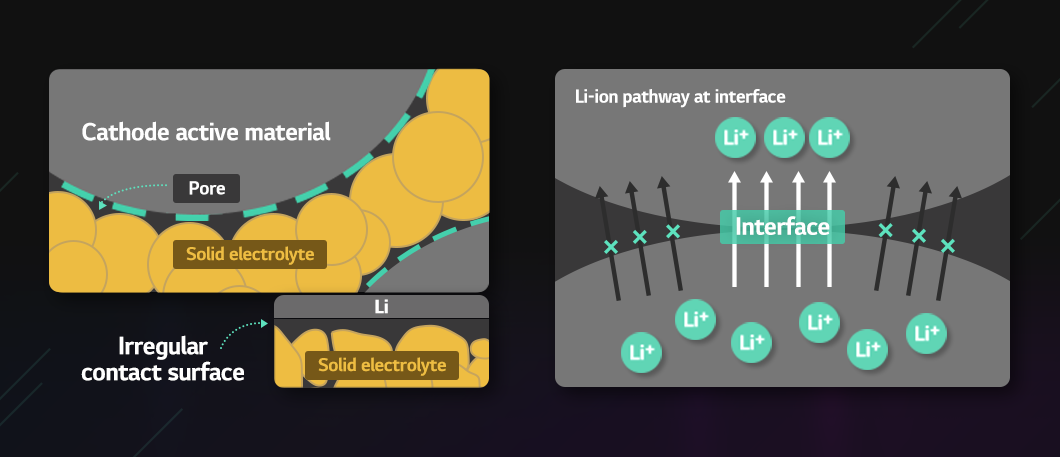
All-solid-state batteries also have challenges to overcome. The first is their “lower ionic conductivity” compared to liquid electrolytes. Solid electrolytes have slower ionic conductivity and lower electrochemical performance at room temperature, which can impact the battery’s output and charging characteristics. Therefore, ongoing research is focused on developing solid electrolyte materials that can improve ionic conductivity.
Another challenge is “high interfacial resistance.” Liquid electrolytes adhere to the electrode because of surface tension, allowing lithium ions to move easily. In contrast, in solid electrolytes, lithium-ion diffusion and the active material’s redox reactions occur only at the contact point between the two materials. If the solid electrolyte is not evenly distributed, the interfacial resistance increases, making lithium-ion movement more difficult. This can negatively impact battery performance, which is why solutions are being developed to maintain solid contact interfaces.
LG Energy Solution’s Efforts to Develop All-Solid-State Batteries
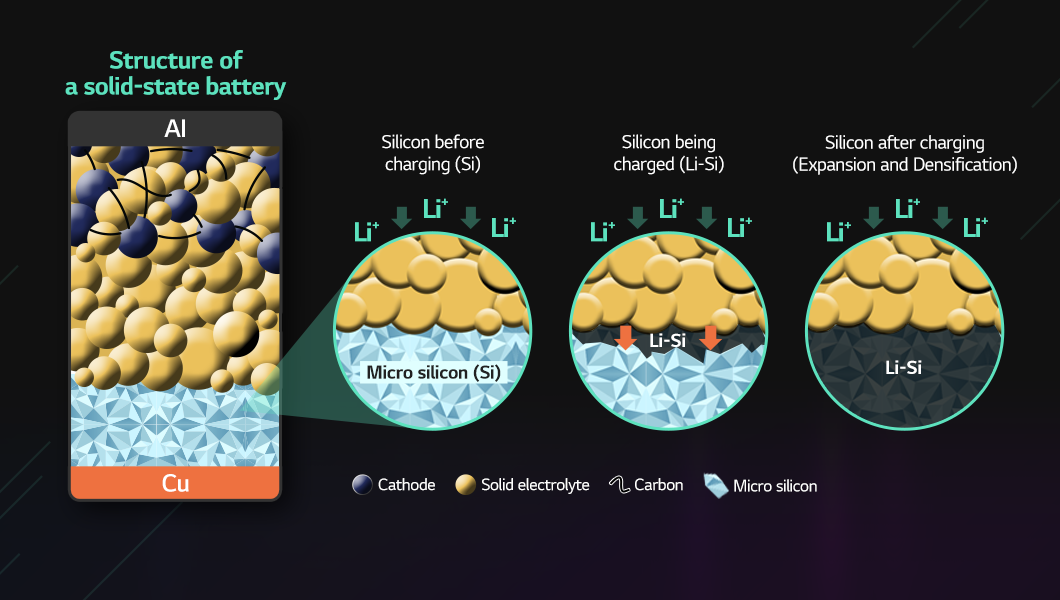
LG Energy Solution is accelerating its research and development of all-solid-state batteries to lead the global battery market. As part of these efforts, LG Energy Solution and the University of California, San Diego (UCSD) jointly developed “long-life all-solid-state battery” technology in 2021, overcoming the technical limitation that required charging temperatures to be at least 60°C. The development made fast charging possible at room temperature (typically 25°C). The research addressed the issue by applying “micro-silicon anode,” of which the particle size is about 5 micrometers. Silicon anodes are known to be challenging to use due to significant volume changes during charging and discharging. Nevertheless, the battery showed innovative results, exceeding 500 charge-discharge cycles while maintaining over 80% residual capacity and improving energy density by more than 40% compared to commercialized lithium-ion batteries. This groundbreaking research was published in the prestigious scientific journal Science (Vol. 373, No. 6562), receiving global recognition.
Today, we explored all-solid-state batteries, which are emerging as the leading next-generation battery. We can see that the arrival of advanced batteries, excelling in safety and performance, is just around the corner. We hope you show great interest in all-solid-state batteries that embody LG Energy Solution’s differentiated technology!